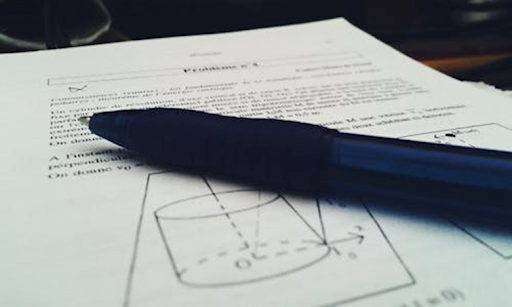
Bell’s Theorem stands as one of the most groundbreaking discoveries in the field of quantum mechanics. This revolutionary theorem challenges deeply rooted classical concepts, particularly the notions of locality and hidden variables that have long been associated with quantum physics. Formulated by physicist John S. Bell in 1964, Bell’s Theorem directly addresses the famous Einstein-Podolsky-Rosen (EPR) paradox, which brought into question the completeness of quantum mechanics as a theoretical framework.
The EPR paradox was originally introduced by Albert Einstein, Boris Podolsky, and Nathan Rosen in 1935, expressing doubts regarding whether quantum mechanics could fully explain the intricacies of the physical universe. Einstein famously referred to the phenomenon of quantum entanglement as “spooky action at a distance.” He found it unsettling that particles separated by vast distances could appear to influence one another instantaneously, a situation that defied the classical notion of locality. Einstein and his colleagues believed that there must be unknown hidden variables—undetected factors that could explain these peculiar behaviors—without violating the fundamental principle of locality, which states that objects are only directly influenced by their immediate surroundings.
Bell’s Theorem Inequality: Testing the Limits of Local Hidden Variables
Bell’s Theorem, however, provides a mathematical framework that challenges this belief, questioning whether hidden variables could indeed account for the predictions made by quantum mechanics. Bell developed Bell’s inequality, a mathematical condition that any theory based on local hidden variables would need to satisfy. In essence, this inequality served as a test to determine whether quantum phenomena could be explained using classical principles of locality and determinism.
Yet, quantum mechanics, in its bizarre and often counterintuitive nature, predicts that this inequality can be violated under certain conditions, such as when dealing with entangled particles. This suggests that no theory based solely on local hidden variables can completely account for or explain the full range of quantum phenomena observed in nature. The experimental violations of Bell’s inequality demonstrate that particles can exhibit correlations that cannot be explained by any local hidden variable theory, thus implying that the universe operates in a fundamentally non-local manner.
The implications of Bell’s Theorem are profound, as they force us to reconsider our most basic understanding of the nature of reality itself. The idea that particles can be connected in such a way that the measurement of one instantaneously affects the other, regardless of the distance between them, defies the classical worldview that dominated science prior to the development of quantum mechanics. Bell’s Theorem challenges us to abandon our intuitive notions of separateness and locality, embracing instead the strange, interconnected reality that quantum mechanics reveals.
This shift in understanding, prompted by Bell’s work, continues to influence not only the field of quantum physics but also extends to other areas of scientific inquiry, philosophy, and even technology. The violation of Bell’s inequality has laid the foundation for advances in quantum computing and quantum cryptography, where the peculiar properties of entanglement are exploited to achieve feats that are impossible under classical frameworks. As physicists continue to explore the implications of Bell’s Theorem, its impact resonates deeply, challenging our concepts of space, time, and the interconnectedness of all things.
Experimental Confirmation
John Clause, in 1972, was the first to experimentally test Bell’s Theorem, performing a landmark experiment that supported quantum mechanics and contradicted the existence of local hidden variables. His experiment used pairs of entangled photons and demonstrated results consistent with quantum mechanics but violated Bell’s inequality.
This violation suggested that particles could be connected over large distances instantaneously, a phenomenon now referred to as “quantum entanglement.”
Other experiments followed, including those conducted by Alain Aspect in the 1980s, which further reinforced Clause’s findings. Aspect’s sophisticated experiments closed some loopholes, increasing confidence that quantum entanglement and non-locality were real phenomena, defying classical expectations.
Implications Beyond Physics
Bell’s Theorem has implications that stretch into philosophy, parapsychology, and technology. Some researchers have speculated that it could provide insights into consciousness, suggesting that quantum entanglement might play a role in mental processes or extrasensory perception.
In technology, quantum non-locality has become the foundation for revolutionary advances such as quantum computing and quantum cryptography. These fields exploit the peculiar properties of entanglement, allowing for faster-than-light information processing and unbreakable encryption methods.
Bell’s Theorem is more than a technical result in quantum mechanics. It signifies a departure from the classical universe understanding, which favored local realism.
The theorem’s experimental validation forces us to reconsider the nature of reality itself, embracing the strange, non-local connections between entangled particles. As physicists continue to explore Bell’s work’s implications, its impact on science, philosophy, and technology remains profound.
If you’re fascinated by Bell’s Theorem’s reflective implications and want to explore the intersection of quantum mechanics, consciousness, and the mysteries of the universe further, Saul-Paul Sirag’s Forays into Hyperspace: From Borneo to Berkeley and Beyond offers a compelling exploration.
Through personal narratives and scientific inquiry, the book takes you on a journey that bridges the worlds of physics, philosophy, and the unknown. Get your copy now and expand your understanding of reality!